Carbon Nanomaterials
Carbon-based nanomaterials, such as graphene, carbon nanotubes, and graphene nanoribbons, hold a pivotal role in nanoscience, particularly in nanoelectronics, due to their exceptional properties. These materials exhibit unique behaviors stemming from electron confinement, which can open a bandgap and generate specific quantum spin states. As a result, carbon nanomaterials are emerging as promising components for next-generation devices. Achieving precise control over their electronic and magnetic properties requires atomic-level structural accuracy. To accomplish this, we employ a bottom-up, on-surface synthesis approach, utilizing the controlled covalent assembly of molecules on metallic templates.
Graphene nanoribbons
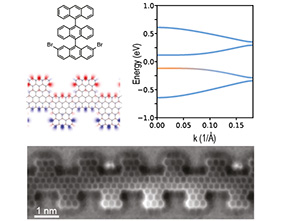
Spatial confinement in graphene nanoribbons (GNRs) induces the formation of a bandgap, which is highly sensitive to the atomic structure of the ribbons. To achieve a bandgap suitable for room-temperature applications, GNRs must be narrower than 5 nm, with their width and edge structure requiring atomic-level precision. Current top-down techniques, such as lithography or etching, cannot meet these exacting demands. In response, we have collaborated with chemists from MPI Mainz and TU Dresden to develop versatile on-surface synthesis methods for the bottom-up fabrication of atomically precise GNRs. These innovative approaches allow us to create GNRs with diverse widths, shapes, and edge structures, enabling us to explore their rich physical and chemical properties through a variety of experimental techniques. Recent advances include the successful fabrication of porphyrin-GNR hybrids, where porphyrin units are covalently attached to the GNRs. This breakthrough paves the way for new applications, such as host-guest systems or sensing devices, utilizing porphyrin properties on a GNR platform.
Magnetic nanographenes
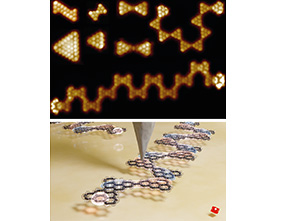
The on-surface synthesis of nanographenes with well-defined shapes enables the creation of molecular building blocks with unpaired electrons, resulting in a net spin localized within a molecular orbital. This presents exciting opportunities for realizing magnetism in a purely carbon-based system. By carefully designing covalent bonds between these magnetic building blocks, we can chemically control spin interactions, unlocking new pathways for manipulating magnetic properties at the molecular level.
This approach allows us to construct quantum spin chains and clusters that exhibit exotic magnetic ground states and excitations, offering insights into fundamental quantum behaviors. Thanks to the atomic precision of our nanostructures, combined with the ability to manipulate single atoms using a scanning probe tip, we can finely tune spin configurations. We characterize these spin systems through spectroscopic techniques such as inelastic electron tunneling spectroscopy (IETS), providing deep insights into their magnetic excitations and interaction dynamics. These advancements lay the foundation for exploring carbon-based quantum magnetic systems, with potential applications in quantum computing and spintronics.
1D / 2D polymers
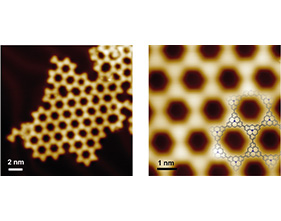
The on-surface synthesis approach can also be harnessed for the fabrication of low-dimensional polymeric materials, including both 1D and 2D structures. Our primary goals are to design covalent 2D networks with tailored electronic and magnetic properties, as well as to create highly precise templates for the selective anchoring of molecular building blocks. These templates offer potential applications in catalysis, molecular electronics, and sensing technologies.
Expanding to 2D magnetic networks presents the exciting possibility of realizing elusive quantum states of matter, such as quantum spin liquids, which can emerge in magnetically frustrated triangular lattices. These systems offer novel opportunities for studying quantum magnetism and may serve as platforms for quantum computing and advanced spintronic devices.
To address the specific challenges associated with both 1D and 2D covalent growth, we are actively exploring new on-surface chemical reactions. This enables us to exert finer control over the reaction sequences and manage the increased reactivity of open-shell molecular building blocks. By advancing these methodologies, we aim to push the boundaries of precision in low-dimensional polymer fabrication, allowing for the synthesis of increasingly complex and functional materials.
-
Share